Rethinking the Land Economy: Keeping 1.5°C in Sight
Land Economy for Sustainability Dialogue Series
This first dialogue took place in early 2017. It explored key elements for a scalable strategy for reducing land-based emissions, drawing on lessons learnt from the past decade on the strategic transformation of the energy sector. It also discussed some of the critical ‘no regret’ interventions that could be scaled up immediately, and identified levers needed to create the preconditions for disruptive change beyond 2030.
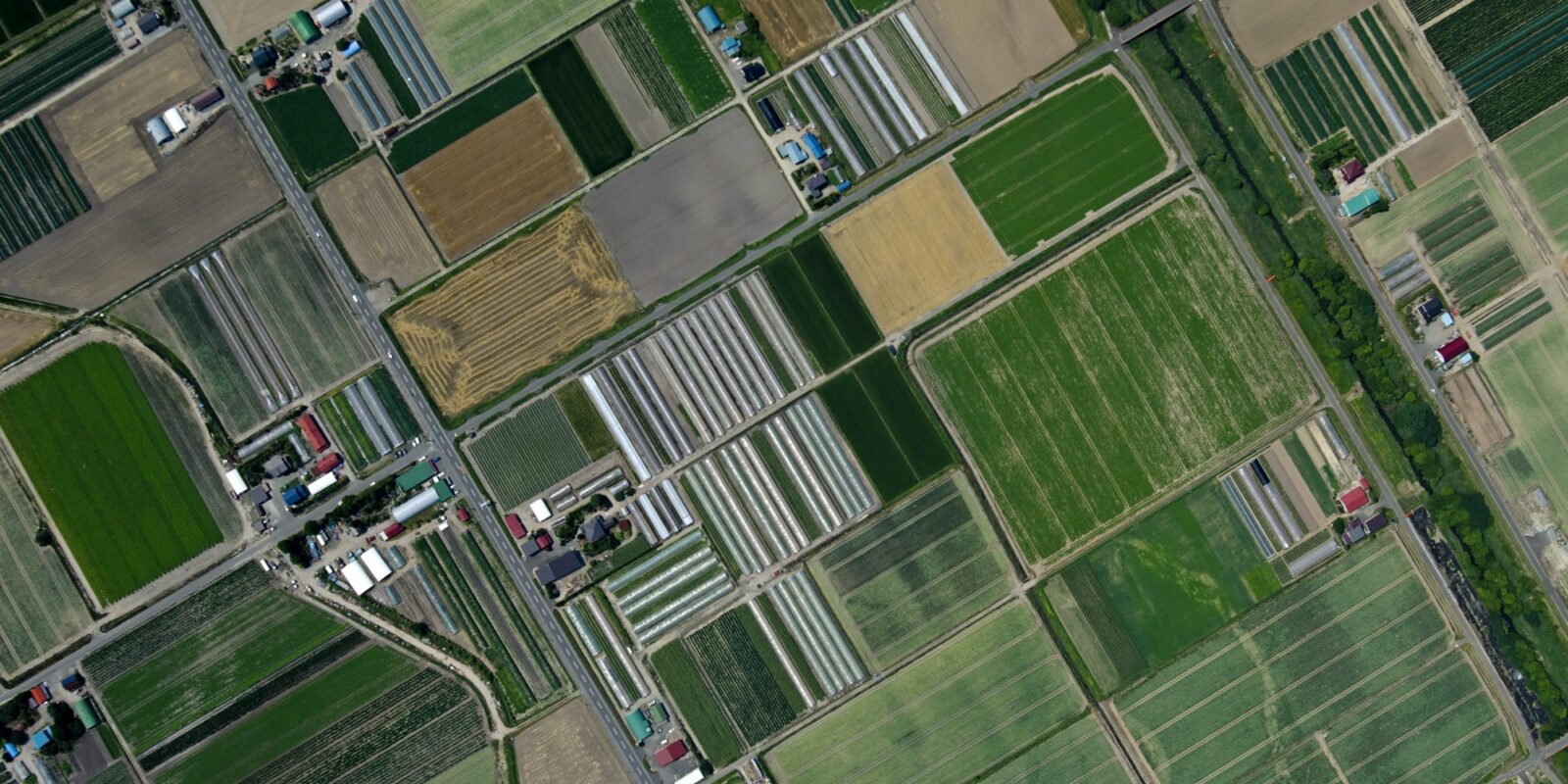
Aerial view of a rice field in Hokkaido, Nakafurano. Image: JTB Photo/UIG via Getty Images
Competition for land is set to intensify. Land provides critical products for humanity, including food, water, energy and a range of other ecosystem services. Over the coming decades, the expectation is that restoration, reforestation, afforestation and other ameliorative measures to bolster carbon sinks will be scaled up to meet climate needs, further increasing competition for finite land resources. For global citizens to continue benefiting from all these services in a climate-changed world, it is imperative to identify targets and viable, plausible pathways to transform the land economy as well as to decouple the provision of some of these essential services from land use.
Anticipated growth in agricultural emissions is sharpening the focus on the future of land use. Land-use change, agriculture, forestry and other land uses together account for 20 to 24 per cent of global anthropogenic greenhouse gases (GHGs).1 While the net emissions from the land sectors have declined since the turn of the century, with reduced deforestation and increased afforestation rates, all the non-CO2 GHGs emissions have risen over the same period.2 Even with efficiency improvements, by 2050 direct emissions from agriculture alone could amount to 45 per cent of annual emissions on a 2°C-consistent pathway. Taking into account emissions from land-use change, this figure could be as high as 70 per cent. Current food-related emissions alone exceed the 1.5°C-compatible global carbon budget for 2050.3
Negative emissions technologies (NETs), many land-based, will be needed to keep global warming to 2°C above pre-industrial levels, let alone to 1.5°C, absent immediate and drastic global emissions reductions. Those with the greatest mitigation potentials typically risk the highest spillover effects, with negative societal, economic and environmental outcomes (including, for example, catalysing indirect land-use change). Additionally, the unproven nature of many NETs at scale, concerns about their reversibility and the associated moral hazard (since their deployment might be used to justify delayed conventional mitigation) and land-use competition call into question any strategies reliant on them to meet climate needs. This risks missing opportunities that are less likely to trigger unintended ripple effects. These include options – such as enhanced weathering, biochar, soil carbon sequestration (including avoiding further peatland degradation) and restoration, reforestation, afforestation – that have substantial mitigation potential, albeit lower than, for example, that of bio-energy with carbon capture and storage (BECCS).
The problem is that many initiatives only focus on aspects of land use in isolation. This silo approach has seen responsibility for agricultural production separated from forest protection across numerous ministries. Atomistic policies that create incentives for a particular use of limited land resources often imply significant trade-offs with other uses. Funding has also concentrated around conservation and avoiding deforestation, though it remains low and insufficient.4 While emissions from deforestation can be partly re-sequestered through reforestation, other ecosystem services – such as biodiversity – that are lost to the same acts of deforestation may never recover.
Key questions remain as how best to identify ‘do no harm’ transformation options that take into account the multitude of ecosystem and societal services provided by land. What makes sense from a carbon-sequestration perspective could compromise food production, for example, and vice versa. Any portfolio of solutions will need to align the interests and priorities of many different actors. This means that national/local targets and pathways for sustainable land use must be consistent with global pathways due to these complexities and trade-offs. At the same time, competition not only for land-use choices but also for policies and policy spaces (which are built on history, specific expertise, mindsets and long-term assumptions) can also stand in the way of rational allocation of resources.
In short, a shared, coherent vision is yet to emerge on the portfolio of viable global and national pathways for transforming the land-based sector, unlike the energy or the transport sectors. Despite the great expectation placed on land-use mitigation pathways, rarely are these given the same level of attention as other sectors like energy. This is in part because some of the solutions, like BECCS, are mired in controversy, while others are often deemed too challenging or costly. That said, there is a clear rationale for coherent, national, cross-sectoral land-use strategies that would help determine the use of a country’s natural capital to provide the appropriate mix of services that fits a government’s assessment of social needs.
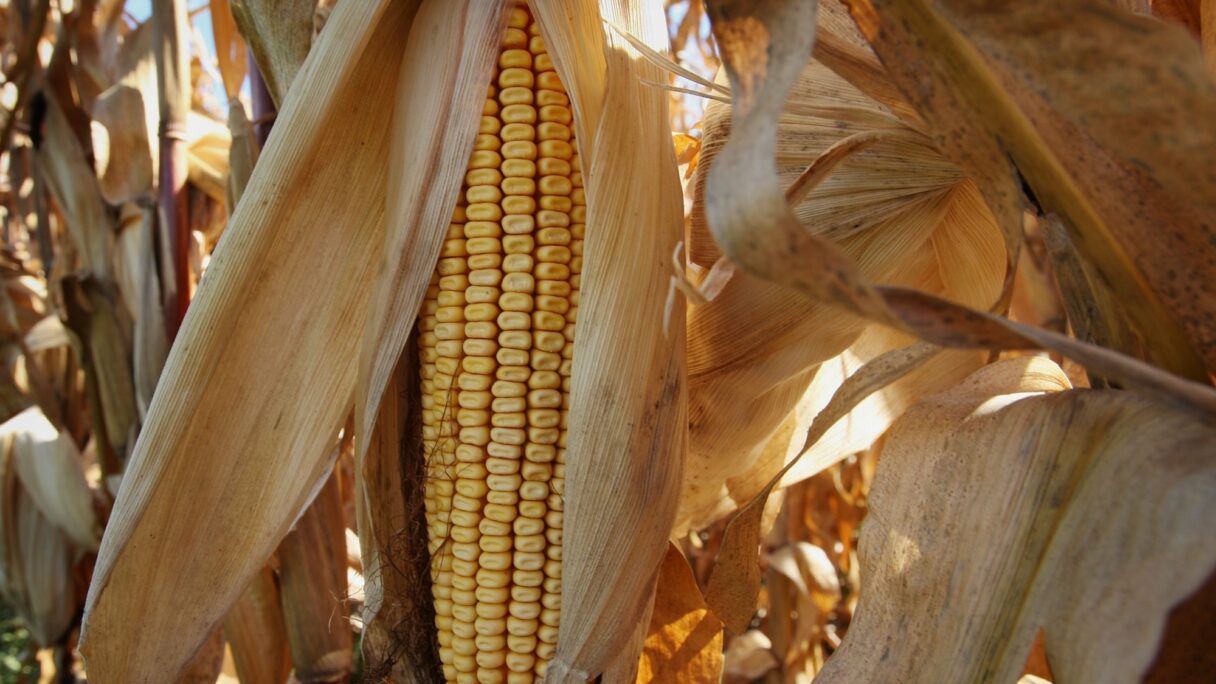
Rising demand for corn and soybeans to make fuel, food and livestock feed has pushed up prices and increased competition for land. Image: Scott Olson/Getty Images
Mitigation opportunities abound, but none is straightforward
The global economic mitigation potential from the land sectors is estimated to be 0.5─10.6 GtCO2eq/yr by 2050 (depending on the carbon price), with notable opportunities from reducing food loss and waste, dietary changes and forest-based mitigation.5 While these discrete options are not necessarily additive, they could complement one another as well as offer health and development benefits.
There is growing awareness of the need for an integrated approach that addresses drivers on the supply and demand sides with respect to land-use change. More integrated approaches are emerging that help reposition nature to sequester more carbon; for example, through land restoration and soil management, bringing also co-benefits in biodiversity, freshwater and clean air. Some progressive businesses are investing in more holistic approaches at landscape level or in integrated land-management approaches at farm level that respond to forest protection and to agricultural production needs. Reducing food waste and loss has resource-efficiency benefits (analogous to energy-efficiency savings in the built environment) that can reduce further production pressure on land.
Forest restoration, reforestation and afforestation are currently the most available and easily deployable NETs to sequester carbon from the atmosphere (despite concerns over the implications for land available for food production as well as their permanence). They can repair abandoned and degraded lands to provide ecosystem services like carbon and water as well as wood products through production forest. For example, if sustainably produced wood-based products could displace fossil-fuel-based ones in building materials, this could double the GHG benefits. Establishing a restoration economy can also create jobs and generate additional economic income.
Technological and other advances are reshaping expectations for systemic transformation in the global food system. These include the adoption of circular-economy practices, leading to reduced waste and more localized production, the introduction of diversified tree and crop species for resilience, breakthroughs in crop science and genome-editing, and the potential of shifting demand patterns around the world. Better monitoring capacity with data management has also helped slow deforestation through cleaner supply chains.
New cultivation methods that could help decouple food production from land use are also emerging. Hydroponics (water-based systems delivering nutrients through fortified solutions), aeroponics (soilless systems using minimal water) and aquaponics (combining aquaculture and hydroponics to cultivate fish and plants in a closed-loop system) are already in use to produce fruit and vegetables. These systems are expected to expand rapidly, which will enable food to be produced in high-demand urban and peri-urban centres. Rapidly falling costs of genome-editing technologies such as CRISPR could be employed on orphan crops (such as sorghum and millet) and on cash crops (like soybean and maize). This could help diversify production and support yield growth in regions where agro-climatic conditions have, until now, stunted expansion and intensification.
Disruption to fertilizer-production models could also be under way. Enabling nitrogen fixation by grain crops could radically reduce dependence on artificial fertilizers. There are microbial-based solutions that use bacteria and fungi as natural fertilizers, insecticides and fungicides. Major agribusiness and pharmaceutical firms – including Bayer, DuPont and Monsanto – as well as organizations such as the Gates Foundation and publicly funded research councils have invested in these options as well as more experimental ones, like genome-editing, to mimic in grains the nitrogen-fixing capacity of legumes. The use of renewable energy could also transform the production landscape of nitrogen fertilizer. Producing ammonia from wind power, for example, could decentralize production and decrease countries’ dependence on a handful of production centres.
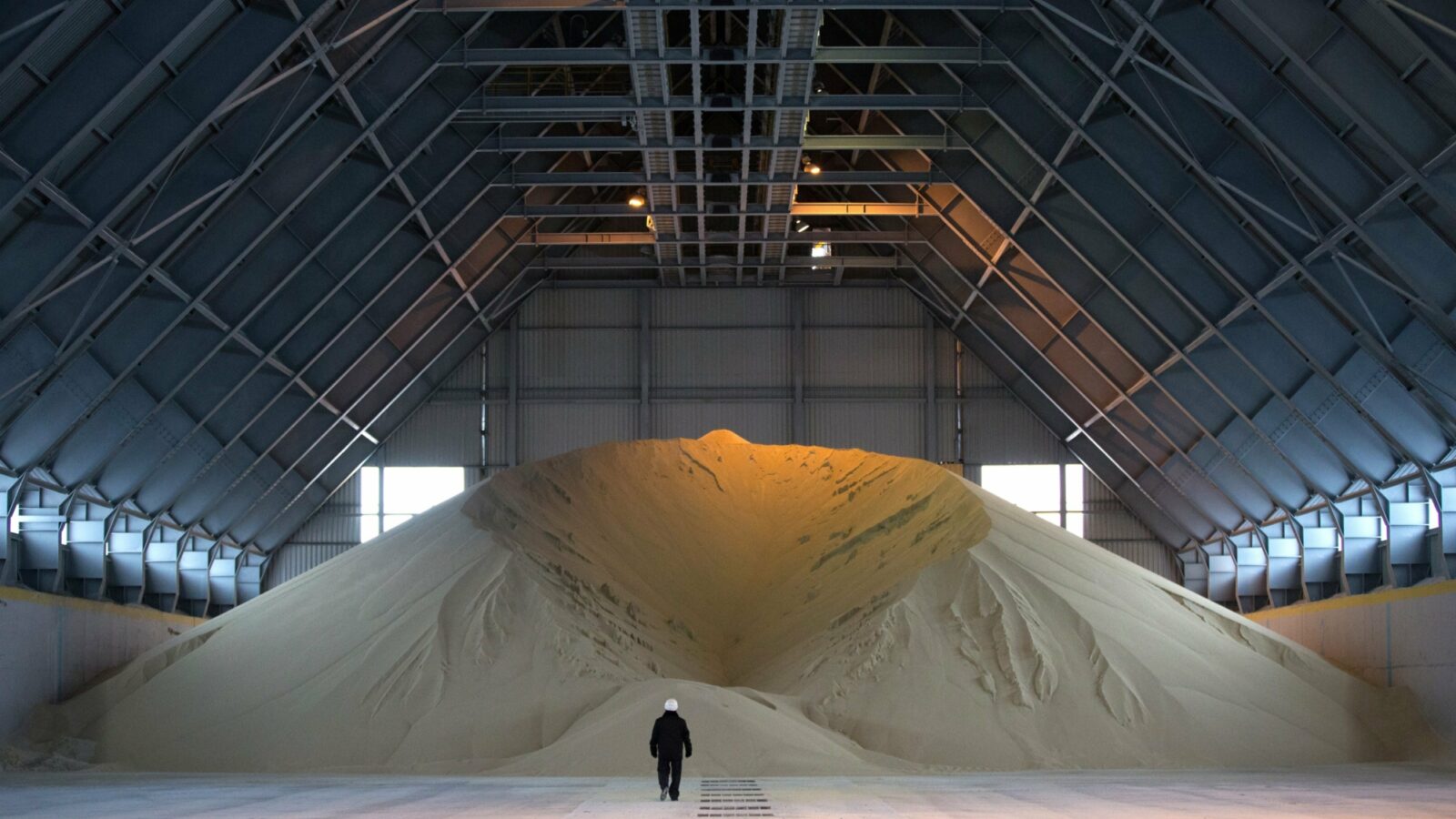
Stockpiles at Europe's largest phosphate fertilizer maker. Disruptions may be on the horizon for the energy-intensive fertilizer production model. Image: Andrey Rudakov/Bloomberg via Getty Images
Media attention around lab-grown burgers and plant-based ‘meat’ products points to a rapidly evolving sector. There is mounting interest in alternative protein products including cultured meat. This new generation of meat alternatives is already on the market in China and the US, and is expected to see fast growth in global markets. Were these products to have a material impact on demand for meat and dairy, the vast volumes of soybean and maize grown and traded to support livestock production could see dramatic declines. Currently, one-third of global cropland is used for livestock feed.6
The agendas on nutrition and climate change are increasingly aligned, especially as regards meat consumption. Should current trends persist, the consumption of meat and dairy products could rise by 76 per cent and 65 per cent respectively by 2050, with China accounting for a significant share of these growths.7 The nutrition transition unfolding across many middle- and low-income countries has driven up demand for grain and fertilizer to support the production of protein-rich and calorie-dense foods, including meat and dairy products. With growing awareness of the negative nutritional outcomes from excessive meat consumption, as well as of the climatic impacts of its production, there has been a sharpened focus on demand-side options to reduce emissions growth from livestock. Changing diets and reducing food loss and waste are highlighted as areas with significant mitigation potential – despite the level of uncertainties around the precise magnitude of mitigation feasible.
Behavioural shift, while uncertain, holds great promise. The success of specific demand-side interventions will be contingent not just on their technical potential but also on their palatability and uptake, as they carry different environmental, nutritional, equity and economic risks. Policy decisions and individual choices about the optimal combination of these various actions and trade-offs are based (whether consciously or not) on underlying behavioural preferences and normative tolerances for these different risks. With $550 billion in fossil-fuel subsidies8 and $580 billion in agricultural subsidies globally, incentives from insurance and subsidy regimes can also be used in helping shift consumption patterns.
Purchasing behaviour is rarely a rational process. Demand is influenced by a mix of consumer desires and needs, commercial profitability considerations (especially among downstream actors), and marketing. Shopping decisions are often made within 30 seconds, while dining choices in restaurants take around 60–90 seconds. Consumer action is influenced by habit, and by what we have seen or remembered during the shopping process.9 The World Resources Institute has identified four potentially effective strategies from research in some 40–50 previous shifts in consumption preferences. These are: (1) minimizing disruption to habits; (2) selling a compelling benefit; (3) maximizing awareness; and (4) evolving social norms.10
Reducing food waste and loss is often presented as one of the best opportunities for emissions savings. Some 24 per cent of food calories produced are lost in the supply chain or wasted during consumption.11 Half of the food loss in China and in the EU is at the consumption stage. Waste also occurs during harvesting, handling and storage. Globally, cutting waste and loss by half would close 22 per cent of the gap between calories available today and those needed by 2050.12
But reducing farm waste may not bring positive land use or environmental outcomes. In many ways, the benefits from waste reduction should be clear. It should help to alleviate pressure on land and forest as well as to reduce fertilizer usage. It should save energy across the distribution chain. It should also reduce methane emissions from landfill. However, if waste reduction ends up increasing production (through efficiency gains) it will not result in demand reduction. In fact, it could contribute to increasing supply capacity as well as consumption, which could take us back to business-as-usual trajectories (unless less efficient production elsewhere is displaced). That nearly 90 per cent of calorie intake comes from eight crops, for example, also adds to the challenge.13 These dilemmas illustrate the complexity in identifying ‘low regret’ options, and point to the need for more specific demand-reduction measures as well as for a strategic and holistic approach.
Another potential game-changer is the deployment of cold-chain logistics and cold storage at scale in developing countries. While not new, these could be effective particularly in remote areas where the risk of weather damage to crop harvests or food stocks is high. As well as reducing waste, refrigeration allows for strategic stockpiling that could help regulate supply and create a buffer in case of shocks.
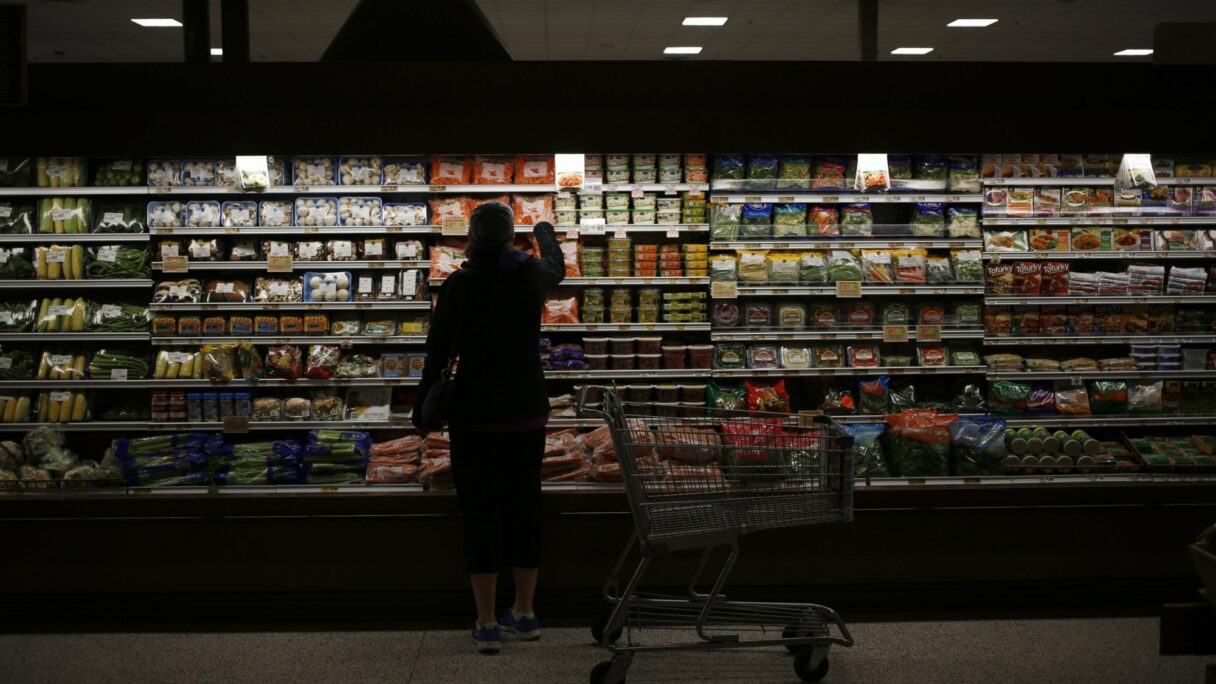
Purchasing behaviour is rarely a rational process. Shopping decisions are often made within just 30 seconds. Image: Luke Sharrett/Bloomberg via Getty Images
Building a common vision through common tools
Transforming land-based sectors into more sustainable development pathways is no mean feat, and is made more complicated by the challenges of identifying the best possible options in any given situation. This is in part because food, water, energy and the other services are interdependent, and any specific interventions could affect the provision of services in complex ways due to the idiosyncrasies of geography and local ecology. In some cases an intervention could bring synergistic benefits, while in others it could result in difficult trade-offs and damaging outcomes.
All 1.5°C-compatible land-use strategies will require due consideration of the system dynamics and ecological limits at different scales (as well as livelihoods and equity issues). This also means taking into account the subjective behaviours and biases of actors within the system that may impede or facilitate progress. Equally, technical efficiency may appear to be a positive instrument but, if the net result is demand rebound, it may trigger less sustainable outcomes.
The efficacy of different options will vary considerably depending on the scale and geographies of deployment, as well as human action and governance. The benefits could also be short-lived or reversible under the wrong circumstances. This means that few interventions are genuinely ‘no regret’ choices. They are more likely to be ‘low regret’ options with narrower bounds of uncertainty, even though they could still bring unintended consequences.
Landscape simulations can help illustrate the different tensions and benefits under different scenarios to determine which are the more efficacious and heterogeneous land uses against a set of optimal boundary conditions and objectives. Instruments like simulations and modelling could contribute to building more judicious and shared visions for future land use, which in turn would enable ‘backcasting’ of potential pathways towards the more desirable outcomes and minimize trade-offs between sustainability, productivity and resilience imperatives.
It is also advisable – and possible – to develop a context-dependent, spatially explicit land-use decision- or discussion-support tool. This exercise could help assemble the jigsaw, based on local interpretations and land-use capability as well as global drivers. The results – in the form of science-based analysis and a transparent platform – can then be used to engage local and global stakeholders and be translated into policy. Such a tool or modelling exercise could also be designed as a ‘what if’. For example, if we used land according to a specific scenario in the future (e.g. minimising GHG or maximizing food), what would be the consequences for biodiversity, water, food and land use at the global, regional and local levels? This exercise could also contribute to trust-building and a better shared understanding of local and global technological needs.
National land-use strategies that are publicly owned and cross-sectoral would also be helpful in illustrating how to optimize the most effective mix of services for governments and stakeholders to meet the range of social, economic and environmental needs. They could also enable the development of government strategies and policies (whether market-based or command-and-control) that could shape and incentivize the needed transitions.
In the absence of more complete information, there are many ‘low regret’ opportunities worth pursuing. These include improving technical efficiency with precision agriculture, changing the incentive structures associated with public and private insurance products and subsidies, diversifying homogenous agriculture, re-carbonizing soils, improving water management through seasonal weather forecasting, and improving the circularity the and horizontal and vertical integrations of supply chains.
For the range of land-use solutions to be effective, it is imperative to pursue not only technological possibilities but also opportunities for behavioural and policy shift. The sector is often mired with deep ideological divides that cloud rational debate and decision-making. Existing policies support incumbents that will seek to maintain the status quo. Behavioural change from production practices and consumption habits need to be understood to unlock the mitigation potential of all the land-use solutions.
This report was written by Bernice Lee, with contributions and comments from Rob Bailey, Tim Benton, Richard King, Laura Wellesley, Graham Wynne and Ana Yang.
This dialogue series, organized by the Hoffmann Centre for Sustainable Resource Economy in collaboration with the Children’s Investment Fund Foundation, seeks to identify key knowledge gaps and to facilitate in-depth, solutions-orientated consideration of the most pressing sustainability challenges facing the global land economy. Bringing together thought leaders from the science and research communities, business organizations, civil society and philanthropy, the dialogue series will also focus on identifying promising opportunities for intervention and building consensus around a strategy for the sustainable and equitable management of land resources.